All published articles of this journal are available on ScienceDirect.
“The Effect of High-Intensity Interval Training (HIIT) on Epigenetic Aging Markers”
Abstract
Background
High-intensity interval Training (HIIT) has emerged as a potent exercise modality with potential impacts on the biological processes of aging. This systematic review aims to explore the effects of HIIT on epigenetic aging markers, including DNA methylation, telomere length, and gene expression related to aging. Additionally, it examines the influence of HIIT on various health biomarkers such as cardiovascular fitness, body composition, and metabolic parameters.
Objective
To synthesize current evidence and identify areas for future research in the application of HIIT as a strategy to modulate epigenetic aging processes and improve overall health outcomes.
Methods
This systematic review followed the PRISMA (Preferred Reporting Items for Systematic Reviews and Meta-Analysis) verification protocol. The study focuses on determining epigenetic markers in High-Intensity Interval Training and the aging clock. The search was conducted in the PubMed, Biomed, ScienceDirect, Scopus, and Google Scholar databases to identify publications published within the past 15 years
Results
The review identified 13 studies that met the inclusion criteria. These studies demonstrated that HIIT interventions, ranging from single acute sessions to 6-month programs, led to significant improvements in epigenetic biomarkers of aging. Key findings included restoration of DNA methylation status in specific genes, increased telomerase activity and telomere length, alterations in mRNA expression related to mitochondrial function, and reductions in transcriptomic age. Additionally, HIIT was associated with improvements in various health markers, including body composition, cardiovascular fitness, and metabolic parameters.
Conclusion
This systematic review provides evidence that HIIT can positively influence epigenetic markers of aging, potentially slowing down or reversing aspects of biological aging. HIIT appears to be an efficient and effective exercise modality for promoting healthy aging at the molecular level. However, further research with larger sample sizes and longer follow-up periods is needed to fully elucidate the long-term effects of HIIT on epigenetic aging markers.
1. INTRODUCTION
High-Intensity Interval Training (HIIT) is an exercise protocol characterized by alternating intervals of vigorous activity and short recovery periods. These exercise bouts are defined as “near maximal” efforts, meaning they are high in intensity but do not reach the level of complete exhaustion; these intensity levels have been quantified through literature using parameters such as maximal oxygen consumption: VO2max (registered as High intensity ≥90), maximal heart rate: HRmax (≥ 77%) or maximal work capacity: Wmax (≥ 80%) [1]. This type of training offers a wide range of modalities by adjusting a minimum of nine factors: work interval intensity and duration, relief interval intensity and duration, exercise modality, number of repetitions, number of series, between-series recovery duration and intensity); any of which has a likely effect on the acute physiological response [1-3].
HIIT has gained popularity due to its consistent ability to trigger physiological changes that enhance health [4, 5]. It improves aerobic and anaerobic capacity, lowers blood pressure, enhances cardiovascular health, increases insulin sensitivity, optimizes cholesterol profiles, and reduces abdominal fat and body weight, all while preserving muscle mass. Additionally, HIT is versatile, time-saving, suitable for various fitness levels and circumstances, accommodating different exercise types like running, cycling, swimming, resistance, and bodyweight training, and can be effectively practiced in group settings [3, 5]. Moreover, the capacity to continue burning calories after a workout in a period known as “EPOC,” or excess post-exercise oxygen consumption, typically lasts approximately two hours. During this time, the body restores itself to its pre-exercise state, and due to the vigorous nature of HIIT workouts, EPOC tends to be slightly elevated, resulting in an additional calorie expenditure of about 6 to 15% [6].
1.1. Standard HIIT Models: From Sprint Intervals to CrossFit
There are numerous types of HIIT based on the change of the variables mentioned before, all preserving the characteristics of High-intensity intervals followed by resting periods. The most commonly used models include Sprint Interval Training (SIT) which involves maximal or supramaximal efforts greater than VO2peak, “all-out” bouts of high-intensity work last short periods of activity (<30 s), separated by relatively long recovery periods between intervals (~4 min); the repeated-sprint training (RST) model, which is characterized by performing a high number of sprints lasting less than 10 seconds interspersed with relatively shorter recoveries (<60 s) [2]. The Tabata model is an exhaustive intermittent training consisting of seven to eight sets of 20 seconds of exercise at an intensity of about 170% of VO2max with a 10 second rest between each bout [7]; another recently studied protocol is the Norwegian 4x4, a structured HIIT method designed to improve cardiovascular health and increase VO2 max effectively, it involves four 4-minute intervals at high intensity (90-95% of your maximum heart rate), each followed by a 3-minute recovery period [8]. CrossFit is a core strength and conditioning program defined as 'constantly varied, high-intensity functional movement'; by leveraging the natural camaraderie, competition, and enjoyment found in its classes, CrossFit aims to optimize physical competence across ten fitness domains: cardiovascular/respiratory endurance, stamina, strength, flexibility, power, speed, coordination, agility, balance, and accuracy. Additionally, to achieve this, CrossFit utilizes various modalities—including weightlifting, gymnastics, and mono-structural exercises—while training across all metabolic pathways. This is accomplished by varying the duration of work and rest intervals and the number of interval repetitions [9-13].
1.2. Aging and Epigenetics
HIIT has emerged as a potent exercise modality for its cardiovascular benefits and potential impact on the biological processes of aging [14]. Recent scientific investigations have begun to unravel the intricate relationship between HIIT and epigenetic markers of aging [15], shedding light on how this form of exercise may influence the mechanisms that control cellular senescence and longevity [16, 17]. Epigenetic markers, which include DNA methylation patterns [18, 19], histone modifications [20, 21], and non-coding RNA expression, play a crucial role in regulating gene expression without altering the underlying DNA sequence [22-24]. These markers are known to change with age and are increasingly recognized as key players in the aging process. The interaction between HIIT and these epigenetic markers presents a fascinating area of study, offering insights into how intense, intermittent exercise might modulate the epigenome to potentially slow down or even reverse certain aspects of biological aging [25-27].
Aging is characterized by the progressive deterioration of various biological functions, spanning from broad processes such as resting metabolism to specific molecular levels, including telomere shortening-induced genomic damage, mitochondrial damage from reactive oxygen species (ROS), accumulation of damaged proteins, and epigenetic alterations [28]. Epigenetics investigates mechanisms capable of modifying gene expression without modifying the genetic sequence, exerting significant influence on aging and frailty by modulating fundamental biological processes via altered gene expression patterns. One extensively researched epigenetic modification is DNA methylation. This process can be seen as two different patterns, either involving the addition or removal of a methyl group at the fifth position of cytosine in DNA. Both patterns have been associated with aging and the appearance of age-related pathologies. Global hypomethylation, for example, is associated with advanced age. Hypomethylation of the amyloid precursor protein (APP) is observed in Alzheimer's disease, type 2 diabetes, and most cancer types. However, certain cancers exhibit the opposite pattern of hypermethylation, as seen in pathogenesis related to insulin-like growth factor-II (IGFII), caspase-8 (CASP8), suppressor of cytokine signaling 1 (SOCS1), RAS association domain family 1A (RASSF1A), adenomatosis polyposis coli (APC), and estrogen receptor 1 (ESR1). Additionally, some inflammatory processes, such as ulcerative colitis, also show hypermethylation [28].PHYSICAL ACTIVITY-INDUCED EPIGENETIC REPROGRAMMING
Overall, physical activity has consistently demonstrated an association with improved health and physiologic markers throughout the literature; for example, the level of VO2max has emerged as a critical biomarker correlated with quality of life, decreased mortality, and improved organ functions [29]. Numerous studies have depicted the decline in physical activity with age, resulting in decreased muscle strength and aerobic capacity, with an approximate 10% reduction in VO2max per decade [30]. However, introducing physical activity to elderly patients can reverse these and other age-related health indicators, including improvements in aerobic and strength conditioning, functional mobility, and cognitive processing abilities [31].
Similarly, physical activity has demonstrated beneficial effects on epigenetic reprogramming, as improving global DNA methylation or showing a higher methylation level, improving the age-dependent decrease in ASC gene, a molecule that mediates IL-1β and IL-18, implying suppression of pro-inflammatory cytokines. Intermittent aerobic exercise can alter mRNA expression and transcription proteins; however, epigenetic adaptations may diminish without regular exercise [32].
The relationship between physical activity and telomere length remains unclear. Some studies have reported associations between physical activity and reduced telomerase activity or increased telomere length compared to baseline. However, other research has found no such relationship, indicating the need for further prospective studies with varying exercise patterns to clarify these findings [33-35].
In summary, aging can be measured at the DNA level through some biomarkers, such as the DNA-methylation changes, the expression of mRNA proteins, and telomere length, which are associated with age-related pathologies. Physical Activity has been associated with improvement in these DNA epigenetic changes. Nevertheless, the aim of this Systematic Review is to show that HIIT provides the most efficient way to reach the goal of reversing or at least pausing the epigenetic clock.
2. MATERIALS AND METHODS
This systematic review adheres to the PRISMA (Preferred Reporting Items for Systematic Reviews and Meta-Analysis) verification protocol (Moher et al., 2009). The study focused on the effect of High-Intensity Interval Training on the Epigenetic markers of aging by searching for articles in the PubMed, ScienceDirect, and Google Scholar databases. Studies were excluded from the review if they were published more than 15 years ago, not found in the databases, or did not meet the search criteria.The study selection process involved two stages. Initially, titles and abstracts of potential studies were screened to include those meeting the criteria and exclude those that did not. In the second stage, the full articles were thoroughly reviewed to further refine inclusion based on the established criteria.
Tailored search strategies were developed for the bibliographic database, focusing on original research articles published within the last 15 years. “The data collection spanned 12 days, from June 6th to June 17th, followed by analysis from June 18th to June 26th”. For all selected studies, the following details were documented: author(s), year of publication, type of study, targeted age group and ethnicity, type of HIIT protocol employed, epigenetic mechanism assessed, and aging parameter evaluated. The author independently reviewed all the articles.
2.1. Eligibility Criteria
2.1.1. Inclusion and Exclusion Criteria
Studies were eligible for inclusion if they met the following criteria: (1) original research articles; (2) implementing a protocol of High-intensity interval training; (3) measuring epigenetic biomarkers; (4) that investigated markers of aging; (5) in humans subjects (6). Excluded studies included those conducted over 15 years ago using non-human models, purely theoretical works, books, and studies that did not align with the review objectives.
2.1.2. Search Strategies
Furthermore, to enhance the search, specific keywords were used, such as “High- Intensity Interval Training” OR “High-Intensity training,” “High Intensity” OR “HIIT,” AND “epigenetic biomarkers” OR “epigenetic clock” OR “epigenetic” AND “aging.”
2.1.3. Identification of Relevant Studies
A comprehensive analysis of epigenetic markers in physical activity was conducted, focusing specifically on HIIT protocols. This analysis followed a bibliographic search protocol adhering to PRISMA guidelines and utilized MeSH (Medical Subject Headings) terms in combination with keywords and Boolean operators (AND, OR). Databases searched included Biomed, PubMed, ScienceDirect, Scopus, Google Scholar, Journal of Sports Science and Medicine, and American Journal of Physical Medicine and Rehabilitation, targeting publications from the past 15 years. The final database search was completed on September 14th, 2024. (Supplementary material)
3. RESULTS
3.1. Study Selection
A systematic search identified 105 studies, 20 in the PubMed electronic database. Following a thorough review of their abstracts, 13 articles were excluded. From ScienceDirect, 15 studies were identified, of which nine were excluded after abstract review, and the remaining did not meet the final criteria upon further examination. From Frontiers, 45 articles were identified, with 12 being excluded and one being ruled out after abstract review, resulting in the inclusion of 33 articles. Neither Scopus nor the journals mentioned above provided any relevant articles. Consequently, this study incorporated 68 references.
3.1.1. Study Characteristics
The following table summarizes the key characteristics of the studies analyzed in this research. These studies were conducted in different countries and published between 2009 and 2024. All selected studies examined the effects of a physical activity protocol in which at least one of the studied arms followed the HIIT protocol, assessing its impact on biomarkers, health markers, and epigenetics, including DNA methylation, telomerase activity, telomere length, mRNA expression, and protein expression related to mitochondrial activity.The studies exhibited a broad spectrum of HIIT durations, ranging from one acute session to interventions lasting up to 6 months. Various forms of training were employed, including running, biking, and cross-training, combining resistance and cardiovascular exercises. Nonetheless, all 13 studies demonstrated significant improvements in health markers and all but one improved in epigenetic biomarkers, reported as reduced biological age, mitigated age-related decline, improved overall biological age, or protection against age-related oxidative stress.
3.1.2. Data Extraction
The following information was extracted for each study in the review: Type of study, sample, age group, ethnicity, type of HIIT employed, epigenetic mechanism studied, other health parameters included in the studies, and the main results observed. Fig. (1).
3.1.3. High-Intensity Interval Training Protocols.
In our data analysis, we identified significant variability in the application of HIIT protocols used to evaluate their effects on epigenetic markers. This variability encompasses several factors: the duration of the intervention, the temporal structure of the exercise bouts, the types of equipment employed, and the methods used to assess and ensure adherence to intensity criteria.
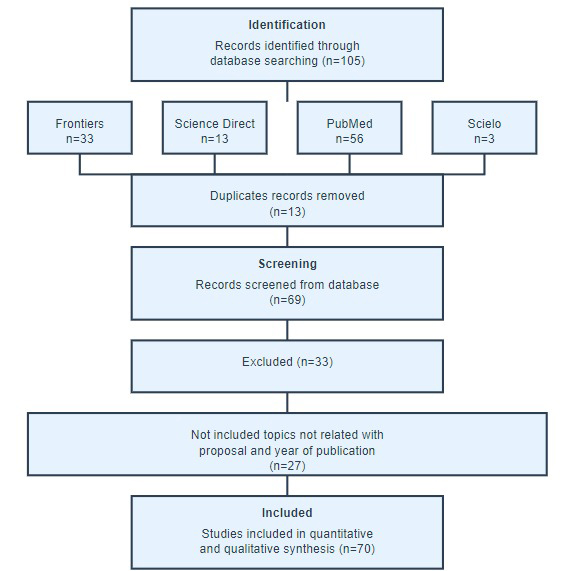
The most notable variability was observed in the duration of the HIIT protocols, which ranged from a single acute session (Barrès R. et al. 2012) to a continuous period of 6 months (Blüher S. et al., 2017; Werner C. et al. 2019), with a 12-week duration being the most frequently utilized timeframe (Robinson M, 2017; Al-Rawaf H. et al. 2023; Streese L. et al., 2020; Jacques M. et al., 2023). Additionally, the time domains of the exercise bouts varied widely, including the most commonly used protocol being 4x4: four sets of four high-intensity rounds interspersed with 3 minutes of rest (Werner C. et al 2019; Streese L. et al, 2020; Robinson M. et al, 2017; Al-Rawaf H. et al 2023) as well as variations involving 1 minute of activity followed by 1 minute of rest, and 3 minutes of activity followed by 1 minute of rest (Williams C. et al, 2021; Hsu C. et al, 2023; Harvey N. et al, 2022; Jacques M. et al, 2023). Rest periods also varied, with some protocols incorporating absolute rest, while others reduced exercise intensity to 50% of heart rate [36, 41].
Further variability was observed in the equipment used to measure variable intensity. Most studies assessed heart rate using different monitoring devices, while one study measured lactate accumulation levels. Exercise modalities also varied, including bikes, ergometers, treadmills, and rowers.
3.1.4. Characteristics of Participants in Studies on the Epigenetic Effects of HIIT
The studies reviewed varied significantly in sample sizes, ranging from as few as 5 participants to more than 500. The age groups studied also differed: Blüher S. et al., 2017 focused on the effects of HIIT in adolescents aged 13 to 18 years, while the majority of studies involved participants aged 18 to 80 years. An important consideration regarding age group differentiation is that the study involving adolescents was the only one that did not demonstrate statistically significant changes in epigenetic biomarkers. The effects observed in this adolescent cohort were primarily limited to improvements in health biomarkers [37].
Gender distribution among the studies was diverse; four studies investigated only male participants (Al-Rawaf H. et al., 2023; Jacques M, 2023; Maasar M. et al., 2021; Harvey N. et al., 2022), one study examined only females (Pashaei Z. et al., 2024), and the remaining studies included both males and females [36, 33, 42, 43].
Geographically, participants were primarily from the United States, Germany, Australia, New Zealand, the United Kingdom, and various European countries, with a predominance of Caucasian subjects. Regarding health status, one study included participants with heart failure and cardiac fibrosis, though they were clinically stable (Hsu C. et al., 2023).
It was observed that stress-induced activation of the epigenetic machinery leads to fibroblast proliferation and subsequent fibrosis. This study [18]by Hsu Chih-Chin et al,2023 reports that HIIT not only improves aerobic capacity in heart failure (HF) patients but also reduces cardiac fibrosis through epigenetic mechanisms, specifically through hypermethylation of the ACADVL gene. These findings suggest that HIIT may be an effective therapeutic strategy to improve cardiac health in patients with heart failure. The results of this study [44]also indicate that both HIIT and MICT can potentially reduce diabetic cardiomyopathy by modulating the expression of miR-206 and its apoptosis-related target genes. However, HIIT appears to be even more effective than MICT in modulating these molecular markers and improving cardiac function in diabetic rats. Similarly, the effects of HIIT extended beyond physiological adaptations to include significant epigenetic changes, resulting in an approximately 10% reduction in cardiac fibrosis. Two other studies focused on obese women and adolescents (Blüher S. et al., 2017; Pashaei Z. et al., 2024), while Streese L. et al., 2020, required participants to have at least two cardiovascular risk factors. The rest of the studies involved participants who were generally healthy [18, 37, 42].
In terms of physical activity levels, Maasar M. et al. 2021 analyzed a cohort of males from a running team. Two studies included moderately trained individuals, and five studies specifically targeted sedentary individuals. The remaining studies did not provide details on the exercise status of the participants. Regardless of the exercise background, positive effects on epigenetic biomarkers were observed [42].
3.1.5. Variability of the Tests used to Demonstrate Epigenetic Changes
Among the studies that investigated RNA as a variable, Linda L. et al. (2012) utilized an app trained to calculate age based on thousands of RNA samples from a previous study, the RNA AGE Calc Shiny App. To achieve this, they paired blood samples collected before and after the intervention to evaluate transcriptomic age (TA) and transcriptomic age acceleration (TA accel = TA – chronological age) using the Transcriptomic Age Prediction (TRAP) tool [ 45, 46]. Robinson M. et al (2017) opt for analysis of mRNA sequencing, protein regulation, and methylation from muscle samples using a kit to analyze transcriptome: TruSeq RNA Sample Prep Kit v2, and processed through the library Infinium 450K array and through Genome Studio [34].
Several methods for assessing DNA methylation were identified in the reviewed studies. Streese L. et al. (2020) examined DNA methylation of the p66Shc promoter by quantifying methylation levels using Methylminer and qPCR. (26) Barrès R. et al. (2012) utilized purified genomic DNA from cultured cells obtained from skeletal muscle biopsies. They processed it to measure CpG methylation within the CCGG sequence using the Luminometric Methylation Assay (LUMA) [38]. Blüher S. et al., 2017 examined the methylation levels of one CpG site using the Pyro Mark Custom Assay [37]. Jacques M. et al. (2023) collected muscle biopsies at baseline and at 4, 8, and 12 weeks of High-Intensity Interval Training (HIIT). They analyzed the epigenetic and proteomic profiles using OMICS techniques, focusing on DNA methylation at approximately 850 CpG sites and assessing around 3,000 proteins [40].
Al-Rawaf H. et al. (2023) assessed melatonin function and lymphocyte apoptosis as epigenetic traits. Melatonin stimulates cell apoptosis via the regulation of many apoptosis facilitators, including mitochondria, cytochrome c, Bcl-2, and the production of reactive oxygen species. They used a colorimetric assay kit to evaluate antioxidant capacity and employed ELISA kits to measure melatonin and cytochrome C oxidase separately. (19) In the same way, in this study conducted by Werner C. et al. (2019), before and after interventions, they isolated mononuclear cells using density gradient centrifugation, then they assessed telomere length using three distinct methods: flow cytometry combined with fluorescence in situ hybridization (FlowFISH), and real-time PCR. Finally, they quantified telomerase activity using the Telomerase Repeat Amplification Protocol [41].
3.1.6. Effects of HIIT on the Epigenetic Markers
3.1.6.1. DNA Methylation
Streese L. et al. (2020) demonstrated that after 12 weeks of HIIT, DNA methylation status was significantly restored in three regions of the gene encoding the mitochondrial adaptor p66Shc in mononuclear cells from peripheral blood, compared to the control group and adjusted for confounders. The p66Shc protein is recognized for its role in promoting apoptosis by regulating oxidative stress and triggering mitochondrial death. Consequently, methylation of this adaptor protein will inhibit its activity [35].
After a single HIIT session, Barrès R. et al. (2012) observed changes in DNA methylation in adult skeletal muscle. However, they noted that these changes were temporary and that other mechanisms, such as the activation of transcription factors and their recruitment to chromatin, are likely involved. Additionally, they found that increased ROS production elevated mRNA expression without affecting promoter methylation. This suggests that DNA methylation alone does not solely govern exercise-induced gene expression, and it may instead act as a selective mechanism to regulate the activation of specific genes [38].
Blüher S. et al., 2017 did not find significant changes before and after the 6 months of HIIT intervention in obese adolescents after measuring methylation levels on one CpG site of the RALBP1 gene that is involved in weight regulation and energy homeostasis and that has been found methylated in other studies. This was the only study that did not demonstrate epigenetic changes after HIIT, and it was also the only one that was conducted on participants younger than 18 years old [37].
Jacques M. et al. (2023) observed alterations in the methylome and, more notably, in the proteome of human skeletal muscle after 12 weeks of intensified exercise training. Over 400 proteins exhibited significant changes, including 26 related to the NADH dehydrogenase complex (Complex I), 10 associated with ATP synthase, and 18 mitochondrial ribosomal proteins. Only one DNA methylation locus significantly correlated with individual responses to exercise. Nonetheless, a detailed analysis of all statistical tests indicated that while widespread changes in the methylome likely occurred, the study's power was insufficient to detect them effectively [40].
3.1.6.2. mRNA
Linda et al. (2012) showed that HIIT could enhance health and longevity by influencing age-related transcriptional processes, thereby reducing transcriptomic age (TA), where the exercise group experienced a notable reduction of 3.59 years and a TAaccel improvement of 7.04 years. This decrease in biological age was accompanied by improvements in other health metrics, as discussed later [46].
Robinson M. et al. (2017) studied three intervention groups: High-Intensity Interval Training (HIIT), Resistance Training (RT), and a Combined (HIIT+RT) regimen. RNA sequencing of muscle biopsies showed significant increases in mRNA expression with HIIT, particularly for mitochondrial transcripts, compared to RT or HIIT+RT. Quantitative proteomics revealed that HIIT led to more pronounced proteomic changes and reversed many age-related alterations. However, only minor changes (less than 10%) in the methylation of DNA promoter regions were observed [34].
3.1.6.3. Telomerase Activity and Telomere Length
Werner C. et al. (2019) examined telomerase activity (TA) and telomere length (TL) in blood mononuclear cells subjected to three types of interventions: High-Intensity Training (IT), Resistance Training (RT), and Aerobic Endurance Training (AET). They found that TL increased, and TA was elevated two- to three-fold in both AET and IT groups, while no such changes were observed in the RT group. These results, when compared to existing literature on telomere-protective phenotypes, underscore the significance of telomerase activity and telomere length in cellular senescence, regenerative capacity, and overall healthy aging [41].
3.1.6.4. Alternative Measurement Approaches
Al-Rawaf et al. (2023) examined the influence of HIIT exercise on the expression levels of melatonin, antioxidant capacity, and related apoptotic markers. Furthermore, the correlation of lymphocyte apoptosis with longer exercise duration was significantly associated with increased serum melatonin following exercise training. This association supports the mechanistic role of melatonin in promoting lymphocyte apoptosis either via the extrinsic mediator pathway or via inhibition of lymphocyte division in the thymus and lymph nodes. Additionally, the correlation between melatonin, lymphocyte apoptosis, TAC, and COX activities significantly supports their role in enhancing physical performance.
Along the same lines, this study [47] investigated the effects of high-intensity interval training (HIIT) in a rat model with features of streptozotocin (STZ)-induced Alzheimer's disease (AD). The results showed that HIIT significantly improved cognitive function, reducing neurodegeneration and neuronal damage. In addition, HIIT facilitated the clearance of β-amyloid (Aβ) and hyperphosphorylated tau from the brain via the glymphatic system to the kidneys. The findings suggest that HIIT promotes the polarisation of astrocytes from a neurotoxic to a neuroprotective phenotype, which is related to a polarised distribution of AQP4, a water channel essential for brain waste removal. In conclusion, HIIT could effectively mitigate AD-associated pathology by regulating astrocyte polarisation and enhancing the clearance of neurotoxic proteins from brain tissue.
Furthermore, these authors showed results of the study [48] found that both high-intensity interval training (HIIT) and moderate-intensity continuous training (MICT) reduced blood glucose levels and improved cardiac function in diabetic rats, with the effects being more pronounced with HIIT. Both protocols decreased miR-206 expression and markers of apoptosis, with more pronounced reductions in the HIIT group. HIIT produced a 20% greater reduction in miR-206 and a nearly 50% greater increase in HSP60 compared to MICT. Both HIIT and MICT improved cardiac performance, with more pronounced changes in the HIIT group. In conclusion, the results indicate that HIIT appears to be even more effective than MICT in modulating these molecular markers and improving cardiac function in diabetic rats.
3.1.6.5. HIIT and other Health Biomarkers Measured
Linda L. et al. (2012) demonstrated a significant reduction in body fat mass, BMI, and visceral fat area over time in the experimental group. Additionally, improvements were observed in the three assessed scales: the Perceived Stress Scale (PSS-10), the Pittsburgh Sleep Quality Index (PSQI), and the Patient Health Questionnaire depression module (PHQ-9), which were evaluated before and after the intervention [33]Al-Rawaf et al. (2023) demonstrated adiposity markers such as BMI, Waist-to-Height Ratio, and CI-index significantly decreased in older adults. Additionally, there were notable improvements in fitness levels (assessed by VO2max) and glycemic control parameters, including Fasting Glucose, HbA1c (%), Fasting Insulin, and serum C-peptide [36]. Robinson M. et al. (2017) showed that HIIT vigorously improved cardio-respiratory fitness, insulin sensitivity, mitochondrial respiration, and fat-free mass (FFM) in both age groups [34]. Streese L. et al. (2020) found that arteriolar widening and venular narrowing were dependent on changes in VO2 max; retinal arteriolar diameters significantly increased, and venular diameters decreased compared to the control group [35]. Following a 6-month (HIT) program in obese adolescents, Blüher S. et al. (2017) evaluated body composition where they observed improvements in body weight, BMI, waist and hip circumferences, body fat percentage, and systolic blood pressure and also, improvement in biochemical markers that included, lipid panel and glycemic panel [38]. Werner C. et al. in 2019 reported an increase in Maximum oxygen uptake (VO2max) [41].
4. DISCUSSION
This research emphasizes the significant advancements in understanding the effects of High-Intensity Interval Training (HIIT) on epigenetic aging markers, achieved through various studies involving human participants. These breakthroughs provide valuable insights that support continuing research in this area. For instance, the study evaluates the impact of HIIT on DNA methylation patterns, telomere length, and gene expression related to aging processes. Moreover, the effects of HIIT on various health biomarkers, such as cardiovascular fitness, body composition, and metabolic parameters, are examined. The review explores HIIT's influences on physiological performance, cellular adaptations, and potential anti-aging effects at the molecular level.
Current studies provide strong evidence for the beneficial effects of High-Intensity Interval Training (HIIT) on epigenetic markers of aging. These findings are consistent with the growing research base suggesting that physical activity, particularly high-intensity exercise, can significantly impact the cellular and molecular biology associated with the aging process. This systematic review by Marriott et al. (2021) examines high-intensity interval training (HIIT) in older adults. Based on 69 investigations involving 3,243 individuals, the review reveals that HIIT significantly improves aerobic capacity (VO2peak) compared to control groups and moderate-intensity continuous training (MCT). However, the results on blood pressure and flow-mediated dilation (FMD) are mixed. The most common protocols include “4 × 4” and the use of cycle ergometers, and HIIT was generally well tolerated, with adherence rates comparable to MCT. However, inconsistent results are observed in body composition and glycemic control, highlighting the need for further studies in clinical populations with chronic diseases to establish optimal protocols and evaluate their impact on the health of older adults. Likewise, this article [49] mentions that it has significant benefits in combating the effects of aging, especially in improving body composition and muscle strength in middle-aged and older adults. The studies analyzed showed that HIIT can help mitigate sarcopenia, a common condition associated with aging that involves the loss of muscle mass and strength. In addition, it was observed that this type of training not only improves muscle strength but also physical function, which is crucial for maintaining quality of life in the elderly population. However, the results of its comparative efficacy with moderate-intensity continuous training remain uncertain, suggesting the need for further research.
Regarding the beneficial effects of physical activity on cellular regeneration, numerous recent studies have examined telomerase activity and telomere length. For example, Werner et al. (2019) conducted a prospective, controlled study demonstrating that resistance training does not increase telomerase activity or telomere length, whereas both aerobic resistance training and high-intensity interval training significantly increased both parameters in healthy, previously inactive individuals. The results suggest that exercise modalities differentially influence aging-related cell biology, highlighting the importance of aerobic exercise for cardiovascular health and the prevention of age-related diseases [50]. Likewise, Mohammadi et al. [51] investigated the impact of manipulating work/rest intervals in high-intensity interval training (HIIT) and detraining on telomerase activity and p53 protein levels in rat heart muscle. The results showed that there were no significant changes in telomerase activity after eight weeks of HIIT, while p53 levels were significantly higher in the HIIT group with long intervals compared to the other groups. However, after a four-week detraining period, p53 levels tended to decrease, suggesting that HIIT does not cause telomere shortening despite the increase in p53 protein. Another study conducted [41] by Werner evaluated the differential effects of endurance, interval, and aerobic training; they found that only aerobic and interval training significantly increased telomerase activity and telomere length in blood mononuclear cells. In comparison, resistance training showed no relevant changes in these parameters. These findings suggest that aerobic exercise modalities are more effective in promoting cellular health and combating aging in previously inactive individuals.
However, some studies show the opposite effect. This study, for example, reviews how regular and intense physical training can prevent DNA damage, telomere shortening, and adverse changes in DNA methylation across the lifespan. Physical activity was positively associated with telomere length and telomerase activity, suggesting that exercise may mitigate biological aging and improve cardiovascular health. Furthermore, it is highlighted that high-intensity training can induce beneficial epigenetic modifications, contributing to healthy aging and reduced risk of age-related diseases [52].
An additional vital aspect to consider is the positive impact of physical activity on mental health across various populations. In children and adolescents, higher physical activity levels during preschool and elementary school are associated with better overall mental health and fewer ADHD symptoms later in life [53]. In adults, recreational physical activity, but not non-recreational activity, is strongly linked to improved mental health outcomes [54]. For older adults, regular physical activity is correlated with lower depression scores [55]. During the COVID-19 pandemic, increased physical activity was associated with enhanced well-being, quality of life, and reduced symptoms of depression, anxiety, and stress across all age groups [56]. As noted in the umbrella review on physical activity and mental health, the protective relationship between physical activity and mental health complications was consistent across age, sex, and geographical regions, with low and moderate-intensity activities showing the most significant protective effects against depression [57]. Linda L. et al. (2012) discussed the effects of HIIT on mental health with parameters such as the Perceived Stress Scale (PSS-10), Pittsburgh Sleep Quality Index (PSQI), and Patient Health Depression module (PHQ-9).
Another essential aspect to highlight is the updated evidence that demonstrates diseases such as cancer, heart failure, and insulin-resistant patients, mainly focused on apparently healthy older adults and/or with one of these conditions [49, 58-60]. Such as are these studies found that it can improve cardiorespiratory fitness in older adults similar or superior to continuous training of moderate intensity [61-63], in addition, these authors agree that Hwang et al., 2016 and Iellamo et al., 2014, the group that performed HIIT showed a significant decrease in insulin resistance, with a 26% reduction, however [58] [59], no changes were observed in the moderate-intensity continuous training (MICT) groups nor in the control group. On the other hand, studies suggest that a high-intensity aquatic exercise program can improve both Bone Mineral Density (BMD) and functional fitness parameters in postmenopausal women [60]. The survey conducted by Neyedli et al. 2020 demonstrates a significant improvement in cognitive flexibility in older adults [49, 58, 61] [62]. It has also been shown that it can improve cardiorespiratory fitness in older adults, and in particular, individuals with cancer may experience improvements in their quality of life and tolerance to treatment through physical activity [64-66]. Finally, the implementation of a well-structured HIIT protocol and attention to appropriate exercise duration is critical to maximizing health benefits and physical performance [67, 1, 68].
In summary, aging can be measured at the DNA level through some biomarkers, such as the DNA-methylation changes or expression of mRNA proteins associated with age-related pathologies. Physical Activity has been associated with improvement in these DNA epigenetic changes. Nevertheless, this Systematic Review aims to show that HIIT provides the most efficient way to reach the goal of reversing or at least pausing the epigenetic clock.
This systematic review highlights the promising potential of High-Intensity Interval Training (HIIT) in modulating epigenetic markers of aging. The studies demonstrated that HIIT can positively influence DNA methylation patterns, telomere length, and gene expression related to aging processes. Moreover, HIIT benefits various health biomarkers, including cardiovascular fitness, body composition, and metabolic parameters. However, the research in this field is still evolving, with some studies showing mixed results, particularly in younger populations.
5. LIMITATIONS
Some studies had similar limitations, which included a limited number of participants, which may affect the generalizability of the results, the relatively short duration (weeks) of the studies, which may not be sufficient to assess long-term effects, the lack of control over other factors that may influence health, the inclusion of targeted populations, the scarcity of non-exercise control protocols may have influenced the response of the studies. In case studies of cancer patients or survivors, the heterogeneity in the populations analyzed may affect the effectiveness of high and/or moderate-intensity training and the impact on quality of life and daily functioning. These limitations suggest the need for future studies with larger samples and longer follow-ups to validate the findings.
CONCLUSION
Despite the limitations of current research, including variability in HIIT protocols and relatively short study durations, the evidence suggests that HIIT could be a time-efficient and effective strategy to combat age-related epigenetic changes and improve overall health. Future research should optimize HIIT protocols for different age groups and health conditions and investigate the long-term impacts on aging processes and age-related diseases. Standardized, long-term studies are needed to fully elucidate the potential of HIIT in modulating the epigenetic aging process.
Furthermore, integrating HIIT into clinical practice and public health initiatives warrants careful consideration. As the population ages globally, interventions that can effectively slow or reverse epigenetic aging could have significant societal impacts. However, safety considerations must be thoroughly addressed, especially for older adults or those with pre-existing conditions. Additionally, future studies should explore the potential synergistic effects of combining HIIT with other lifestyle interventions, such as nutrition or stress reduction techniques, to maximize its impact on epigenetic aging markers and overall health outcomes.
AUTHORS’ CONTRIBUTION
“AOM, FGB JCO contributed to the conception and design of the study and organized the database. JCO performed the statistical analysis. JCO wrote the first draft of the manuscript. AOM, FGB, and JCO wrote sections of the manuscript. All authors contributed to manuscript revision, read, and approved the submitted version.”
LIST OF ABBREVIATIONS
HIIT | = High-Intensity Interval Training |
HIT | = High-Intensity Training |
VO2max | = Maximal Oxygen Consumption |
HRmax | = Maximal Heart Rate |
Wmax | = Maximal Work Capacity |
SIT | = Sprint Interval Training |
RST | = Repeated Sprint Training |
EPOC | = Excess Post-Exercise Oxygen Consumption |
RT | = Resistance Training |
AET | = Aerobic Endurance Training |
BMI | = Body Mass Index |
FFM | = Fat-Free Mass |
PSS-10 | = Perceived Stress Scale |
PSQI | = Pittsburgh Sleep Quality Index |
PHQ-9 | = Patient Health Depression Module |
TA | = Transcriptomic Age |
TRAP | = Transcriptomic Age Prediction |
LUMA | = Luminometric Methylation Assay |
FlowFISH | = Flow Cytometry combined with Fluorescence In Situ Hybridization |
PCR | = Polymerase Chain Reaction |
ROS | = Reactive Oxygen Species |
TAC | = Total Antioxidant Capacity |
COX | = Cytochrome C Oxidase |
AD | = Alzheimer's Disease |
Aβ | = Beta-Amyloid |
STZ | = Streptozotocin |
AQP4 | = Aquaporin-4 |
MICT | = Moderate-Intensity Continuous Training |
HSP60 | = Heat Shock Protein 60 |
FMD | = Flow-Mediated Dilation |
MCT | = Moderate-Intensity Continuous Training |
DNA | = Deoxyribonucleic Acid |
RNA | = Ribonucleic Acid |
mRNA | = Messenger RNA |
CpG | = Cytosine-Phosphate-Guanine |
ELISA | = Enzyme-Linked Immunosorbent Assay |
BMD | = Bone Mineral Density |
AVAILABILITY OF DATA AND MATERIAL
All the data and supporting information are provided in the article
ACKNOWLEDGEMENTS
JCO wishes to acknowledge the support of his family and encouragement through his journey.