All published articles of this journal are available on ScienceDirect.
Association Between Vitamin D Deficiency and Tuberculosis among HIV-Negative Individuals: A Systematic Review & Meta-Analysis
Abstract
Background and Objective
Tuberculosis is an infectious disease mainly caused by Mycobacterium tuberculosis (MTB). It is associated with several factors, with vitamin D deficiency being a focus of current research. This systematic review aims to compare plasma vitamin D levels between TB cases and controls and examine the association between vitamin D deficiency and TB risk in HIV-negative participants through meta-analysis.
Methods
This study, registered with PROSPERO (CRD42024518012) and conducted per PRISMA guidelines, analyzed the association between vitamin D deficiency and tuberculosis. Searches were conducted in PubMed/Medline, Scopus, ScienceDirect, and Web of Science for studies up to March 8, 2024. Two independent reviewers screened articles, extracted data, and assessed bias. A random-effects model and R software were used for statistical analyses, with significance set at P < 0.05.
Results
We identified 2168 articles, and only 9 articles meeting the inclusion criteria were retained for our systematic review and meta-analysis. Sample sizes ranged from 33 to 520 participants. We found a mean difference of -6.58 ng/ml in 25(OH)D levels, indicating consistently lower levels in TB patients compared to controls (p<0.0001) and an increased risk of TB associated with VDD with a pooled OR of 2.11 (95% CI: 1.50 to 2.98), p=0.09.
Conclusion
This study highlights lower 25(OH)D levels in TB patients and the association between vitamin D deficiency and increased TB risk, emphasizing the need for further research, standardized clinical trials, and preventive supplementation protocols.
1. INTRODUCTION
Tuberculosis (TB) is an infectious disease caused mainly by Mycobacterium tuberculosis (MTB) [1]. The disease is commonly spread through the air when individuals with active TB cough or sneeze, dispersing droplets that carry the MTB [2, 3]. While pulmonary tuberculosis (PTB), which affects the lungs, is the most prevalent form, TB can also impact other organs, resulting in extrapulmonary tuberculosis (ETB) [1]. Currently, TB remains the second leading cause of death from infectious diseases worldwide after COVID-19 (surpassing HIV/AIDS) [1]. In 2022, 10.6 million people in the world contracted TB, and 1.3 million died from it [1]. The WHO Southeast Asia Region, the African Region, and the Western Pacific Region had the highest incidences, with 46%, 23%, and 18%, respectively [4]. Several factors associated with tuberculosis have been identified, including HIV infection, smoking [1], comorbidities [5], alcohol abuse, and poor socio-economic conditions, such as low income, lack of education, malnutrition, unsuitable housing, and other social determinants of TB [6]. Additionally, current studies are exploring potential contributing factors, particularly vitamin D deficiency (VDD) [7-10]. Vitamin D or 25-hydroxyvitamin D (25[OH]D) is defined as a fat-soluble prohormone steroid known to be involved in phosphocalcic metabolism [11], the immune response against microbial pathogens and many other metabolic disorders [12-14]. It is mainly synthesized by the skin's exposure to ultraviolet sunlight but can also be provided by diet and supplements [13-17]. Several definitions are used to assess plasma levels of 25-hydroxyvitamin D (25(OH)D), and recommendations differ regarding the threshold for defining vitamin D deficiency [15]. According to Giustina et al., vitamin D deficiency is defined as levels below 12 ng/mL (30 nmol/L) [15], whereas other studies define it as a 25(OH)D level below 20 ng/mL (50 nmol/L) [16, 18]. Currently, vitamin D deficiency is a major public health problem in many countries and even continents. A recent meta-analysis of 7.9 million participants estimated that 15.7% of participants had serum 25-hydroxyvitamin D levels below 30 nmol/L, and 47.9% had levels below 50 nmol/L [19]. The same study highlighted that vitamin D deficiency could contribute to an increased global burden of disease. This situation creates the conditions for contracting tuberculosis, which in turn worsens the nutritional status, leading to a vicious cycle [20]. Several observational studies have reported significantly lower vitamin D levels in TB patients compared to their controls [7, 8, 21-23] and demonstrated an association between vitamin D deficiency (VDD) and tuberculosis [24-26, 11]. However, other observational studies have reported contrasting results or inconclusive results, with no significant association between VDD and TB [27, 28]. Even recent meta-analyses have yielded inconsistent results. Cao et al. found no association between serum vitamin D levels and the incidence of LTBI, nor did they find that higher serum vitamin D levels conferred protection against LTBI [29]. In contrast, Acen et al. demonstrated that pulmonary tuberculosis is significantly associated with hypovitaminosis D [30]. In view of the contrasting and inconclusive results concerning the association between VDD and TB, it is evident that, although some studies suggest a possible association between these two factors, the number of studies providing conclusive evidence remains limited. Furthermore, many existing studies fail to address methodological biases that could affect their results, such as omitting HIV status, which is often not verified or reported in studies exploring this association. This systematic review aims to compare plasma vitamin D levels between TB cases and controls and to establish the association between vitamin D deficiency and the risk of developing TB by evaluating primary studies that exclusively included HIV-negative participants and applying quantitative analysis through meta-analysis.
2. METHODS
This study was conducted according to the preferred reporting items for systematic reviews and meta-analyses (PRISMA) guidelines [31] and was registered with the International Prospective Register of Systematic Reviews (PROSPERO) under number CRD420245 18012 (supplementary data).
2.1. Data Sources and Inclusion Criteria
The following databases were searched: PubMed/Medline, Scopus, ScienceDirect, and Web of Science for published studies testing the association between Vitamin D Deficiency and tuberculosis from inception to 8 March 2024. The search strategy utilized a combination of medical subject headings (MeSH) terms and keywords to ensure a thorough and broad search. The key terms included “vitamin D,” “vitamin D deficiency,” “25-hydroxyvitamin D”, “1,25-dihydroxy vitamin D”, “25(OH)D”, and “tuberculosis,” both as MeSH terms and keywords. The detailed search strategy is provided in the supplementary data. All observational studies (cross-sectional, cohort, and case-control studies) of tuberculosis patients examining the association between VDD and TB, regardless of sample size or age, published in English or French, were included. Case series, case reports, studies without a comparison group, studies with unavailable data, studies that did not mention HIV status, clinical trials, systematic reviews, meta-analyses, and studies involving participants diagnosed with conditions known to affect Vitamin D levels, as well as those with human immunodeficiency virus (HIV) or acquired immune deficiency syndrome (AIDS) were excluded.
2.2. Data Extraction
The data was compiled, and duplicates were removed. Two independent reviewers (AO and SB) selected articles based on predetermined criteria. The reviewers assessed titles, abstracts, and full-text articles for inclusion. Disagreements regarding inclusion are addressed and resolved through consultation with a third reviewer. The following data were extracted from the articles: author, year, country, type of study, target population, the method used to assess serum 25(OH)D levels and the cut-off value for vitamin D deficiency and the association of vitamin D, including epidemiological measures such as relative risk, odds ratio, and their respective 95% confidence intervals.
2.3. Quality Assessment
The two reviewers (AO and SB) independently assessed the risk of bias for each included study using the Newcastle-Ottawa quality assessment Scale [32]. This scale evaluates studies based on three domains: (1) selection of subjects, (2) comparability of groups, and (3) assessment of outcomes or exposures. Each study was assigned a score ranging from 0 to 9, with quality categorized as low (0-3), moderate (4-6), or high (7-9) [29-33]. A third examiner resolved potential disagreements.
2.4. Definition of Outcomes
In this study, vitamin D deficiency was defined as serum 25-OH-D below 20 ng/mL (50 nmol/L). This threshold is based on widely accepted clinical guidelines [15, 16]. To convert 25-OH-D concentration values from the conventional system (ng/mL) to the international system of units (nmol / L), we multiplied by the factor 2.496 [34].
2.5. Statistical Analysis
We performed a meta-analysis of the mean difference (MD) between patient and control groups. For studies that presented results as medians and ranges, these data were converted to means and standard deviations using the Hozo method [35]. In our study, vitamin D deficiency was defined as a concentration below 20 ng/ml [15]. Analyses were performed using a random-effects model to account for heterogeneity between studies. For odds ratios (ORs), we also employed a random-effects model to obtain an overall estimate of the association between vitamin D deficiency and tuberculosis. ORs and RRs were treated as ORs, and all data from the included studies were converted to log (OR) and standard error (SE). Heterogeneity was assessed using Cochran's Q statistic and the I2 statistic. An I2 value greater than 50% was considered indicative of substantial heterogeneity. The Tau2 statistic was used to estimate the variance between studies in the random-effects model. Publication bias was examined visually using funnel plots and statistically using the Egger test. An asymmetrical funnel plot and a p-value < 0.05 for the Egger test were considered indicative of potential publication bias. All statistical analyses were performed using the R software, specifically the “meta” and “metafor” packages. Statistical significance was set at P < 0.05.
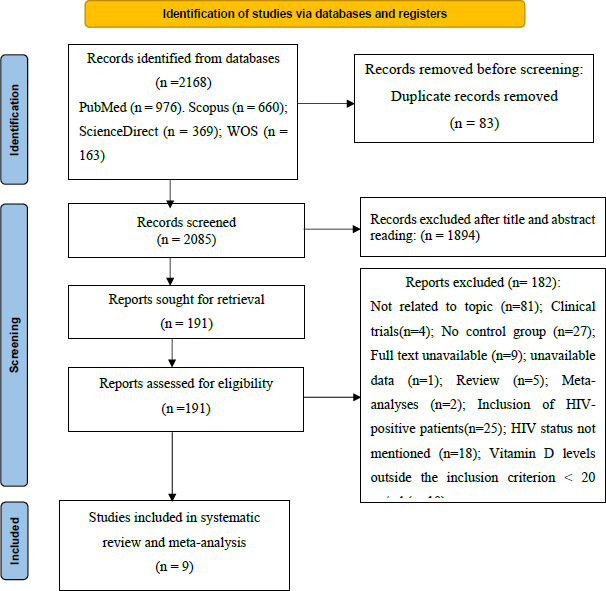
3. RESULTS
3.1. Results of Study Search
A bibliographic search was carried out in several electronic databases, including PubMed, Scopus, ScienceDirect, and Web of Science, identifying a total of 2168 articles published from the inception of the database until March 8, 2024. An Excel spreadsheet was used to detect duplicates, leading to the removal of 83 articles, leaving 2085 records to be examined. After the evaluation of titles and abstracts, 1894 articles were excluded. The remaining 191 articles were reviewed in full text, of which 182 were excluded for the following reasons: not related to topic (n = 81), clinical trials (n = 4), no control group (n = 27), full text unavailable (n = 9), unavailable data (n=1), review (n = 5), meta-analysis (n = 2), inclusion of HIV-positive patients (n = 25), HIV status not mentioned (n = 18), and vitamin D levels not meeting inclusion criteria of less than 20 ng/ml (n = 10). Finally, 9 articles meeting the inclusion criteria were retained for our systematic review and meta-analysis (Fig. 1).
Author (Ref.) |
Country | Study Design |
Study Population |
Population |
Age, Years Mean ± SD/ Median (Range) |
Method of Measuring Vitamin D |
Vit D ng/ml (25OH D) |
Association TB & VDD | |||
---|---|---|---|---|---|---|---|---|---|---|---|
Case | Control | Case | Control | Case | Control | ||||||
Jaimni (2021) | India | Case-control | PTB patients/ healthy controls |
50 | 50 | NA | NA | ECLIA | 19(7.75-27.25) | 25(19.75, 32) | Yes |
Wang (2019) | China | cross-sectional | PTB patients/ healthy controls | 299 | 91 | 45.14±16.45 | 47.45± 9.24 | RIA | 6.50±0.22 | 17.21±0.14 | Yes |
Workineh (2017) | Ethiopia | cross-sectional | TB patients/ HHC/Controls | 126 | 70 | 29.8±11.9 | 27.3 ± 7.6 | ELISA | 30.1±19.3 | 38.5 ± 20.9 | Yes |
Balcells (2017) | Chile | cross-sectional | PTB patients/ HHC/controls | 107 | 31 | 37(29–53) | 32 (26–48) | LC-MS/MS | 11.7 | 23.4 | Yes |
Belyaeva (2017) | Russia | cross-sectional | PTB patients / healthy donors | 33 | 41 | 22.9(17–33) | 31.5(17–56) | ELISA | 11.7±1.8 | 19.3 ± 1.4 | Yes |
Jongwon (2016) |
South Korea | Case-control | TB patients/ controls | 152 | 137 | 48(18-80) | 45 (21-80) | LC-MS/MS | 10.6(0.49-52.3) | 19.3(6.2-60.5) | Yes |
Junaid (2016) | Pakistan | Case–control | PTB patients/ controls | 260 | 112 | 31.6 ± 10.5 | 30.6 ± 9.3 | ELISA | 27.3 | 43.3 | OR =1.87 (1.15 - 3.04) |
Arnedo-Pena (2014) | Spain | cohort | Contacts of PTB patients | 3 | 520 | 42.3±24 | 38.0± 14.0 | ECLIA | 13.7±6.3 | 25.7±12.7 | HR=0.89 (0.80–0.99) |
Hong (2014) | South Korea | Case-control | TB patients/ controls | 94 | 282 | 35.4±15.5 | 35.4 ± 15.2 | RIA | 9.86 (7.19–14.15) | 16.03(12.38–20.30) | OR=2.64 (1.33–5.22) |
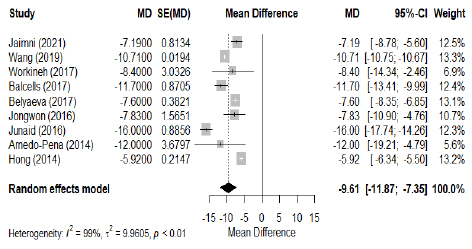
3.2. Characteristics of the Studies
Table 1 presents the characteristics of the nine included studies [7, 8, 21-25, 36, 37]. These studies come from various geographical regions, with the majority based in Asia (India, China, South Korea, Pakistan), two from Europe (Spain, Russia), one from Africa (Ethiopia), and one from South America (Chile). Among the included studies, four are cross-sectional studies [8, 21, 23, 36], four are case-control studies [7, 22, 24, 25], and one is a cohort study [37]. Sample sizes ranged from 33 to 520 participants, with the largest sample size observed in a Spanish cohort study [37]. The average age of participants varied considerably between studies. On average, the age of cases is around 36.28 years, while that of controls is 36.6 years, with a mean age variation of 0.32 years between the two groups. Some studies provide medians instead of means [22, 23, 36], with median values ranging from 22.9 years [36] to 48 years [22]. It should be noted that in one study, age data were not provided [7]. Regarding vitamin D measurement methods, nine studies used four main techniques to measure vitamin D levels. Of these, enzyme-linked immunosorbent assay (ELISA) was used in three studies [21, 36, 24], while liquid chromatography with tandem mass spectrometry (LC-MS/MS) was utilized in two studies [22, 23]. The radio immunoassay (RIA) [8, 25] and electro chemilumine scence immunoassay (ECLIA) techniques were each used in two studies [7, 37]. Vitamin D levels in cases ranged from 6.50 to 30.1 ng/ml, while in controls, they ranged from 16.03 to 43.3 ng/ml. Tree studies [7, 22, 25] provided medians (range) for vitamin D levels, whereas the remaining studies presented means ± standard deviation [8, 21, 23, 24, 36, 37]. All studies reported a significant association between vitamin D deficiency and tuberculosis, with vitamin D levels consistently lower in tuberculosis patients compared with healthy controls. Six studies reported a significant difference in mean vitamin D levels between the two groups [7, 8, 21-23, 36], while two case-control studies reported a significant association between vitamin D deficiency and active tuberculosis [24, 25]. Additionally, one cohort study identified an inverse association between Vitamin D status and tuberculosis incidence [37]. The quality assessment of the included studies was classified as high, with a score between 8 and 9 on the Newcastle-ottawa scale (supplementary data).
3.3. Meta-analysis
The meta-analysis, performed using a random effects model, revealed significant differences in 25(OH)D levels between tuberculosis (TB) patients and controls. In all the included studies (Fig. 2), TB patients had an average reduction in 25(OH)D levels of -9.61 ng/ml (95% CI: -11.87 to -7.35), with considerable heterogeneity (I2=99%, p<0.01), suggesting a substantial variation between studies. To mitigate this heterogeneity, a sub-group analysis of four studies was carried out (Fig. 3), showing a mean difference in 25(OH)D levels of -6.58 ng/ml (95% CI: -7.71 to -5.44) with moderate heterogeneity (I2=29%, p=0.24). This sub-analysis confirms that 25(OH)D levels were systematically lower in patients with tuberculosis. Meanwhile, examination of the association between VDD and TB risk, expressed as odds ratio (OR), shows a significant association, with a pooled OR of 2.11 (95% CI: 1.50 to 2.98, Z = 4.26, p < 0.001; I2=59%, p=0.09) (Fig. 4). These results highlight the association between VDD and an increased risk of developing tuberculosis.
3.4. Publication Bias
Funnel plots and Egger tests were used to assess publication bias. The funnel plots were examined visually for any asymmetries, and statistical tests were performed to assess the presence of bias. The results of the Egger tests showed p-values of 0.2908, 0.0612, and 0.5980, indicating varying levels of evidence of asymmetry. Specifically, the first and third tests revealed no significant asymmetry, suggesting that publication bias is unlikely. The second test indicated a slight tendency towards asymmetry, but this was not statistically significant. Overall, these findings confirm that publication bias does not affect the results of this meta-analysis (supplementary data).
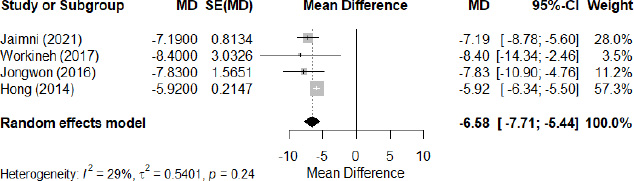
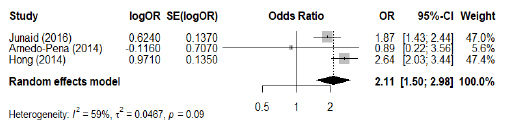
4. DISCUSSION
In this meta-analysis, we included studies that involved HIV-negative participants to explore the association between vitamin D deficiency (< 20 ng/mL) [18] and tuberculosis. our finding revealed significant differences in 25(OH)D levels between the TB group and controls, with vitamin D concentrations being notably lower on average in the TB group. Vitamin D deficiency was observed in four [8, 25, 36, 22] of the nine studies included in the control group and seven of the nine [7, 8, 22, 23, 25, 36, 37] studies included in the TB group, indicating a significantly higher prevalence of VDD in TB patients compared with controls. All studies [7, 8, 21-25, 36, 37] reported negative mean differences (MD) between the groups, with an overall MD of -9.6097 ng/ml (95% CI: -11.87 to -7.35). A sub-group analysis showed a mean difference in 25(OH)D levels of -6.58 ng/ml (95% CI: -7.71 to -5.44) with moderate heterogeneity (I2=29%, p=0.24), consistently indicating lower 25(OH)D levels in TB patients (p<0.0001). Acen et al. also reported similar results, finding that TB patients had significantly lower vitamin D levels than those without TB (p < 0.01). Their meta-analysis estimated the standardized mean difference (SMD) in vitamin D levels, revealing an overall pooled effect of SMD -0.625 (95% CI [-0.784, -0.466], (p < 0.001) [30]. Similarly, Huang et al. conducted a meta-analysis of 25 studies involving 3599 TB cases and 3063 controls, revealing significantly lower 25(OH)D levels in TB patients than in controls (MD = -13.05; 95% CI [-19.02, -7.08]; p < 0.0001) [38]. Several other recent studies, including both reviews and primary research, have yielded similar results [39-41, 9]. This consistent observation across studies underscores the main role of vitamin D in the immune response against microbial pathogens, particularly those caused by Mycobacterium tuberculosis [13, 14, 42]. Furthermore, in the presence of vitamin D, monocytes and macrophages produce antimicrobial peptides, such as cathelicidin, which enhances their ability to eliminate bacteria more effectively. In addition, vitamin D regulates the response of type 1 T helper lymphocytes (Th1), crucial for the production of interferon-gamma (IFN-γ), a key cytokine in TB control [13, 14, 43-45]. Moreover, our findings highlight the significant association between VDD and an increased risk of developing tuberculosis with a pooled OR of 2.11 (95% CI: 1.50 to 2.98, Z = 4.26, p < 0.001; I2=59%, p=0.09). Various studies have suggested an association between VDD and tuberculosis. Davies et al. were the first to establish this link in 1985 [46]. Over the years, several studies have identified a significant association between VDD and tuberculosis [26, 9, 47, 10, 39]. A recent meta-analysis by Kafle et al. [40] and Huang et al. [48] reported similar results with (OR=3.23, 95% CI = [1.91–5.45], p < 0.0001) and (OR = 4.26; 95% CI =[2.48, 7.30], p <0.00001) respectively. Even in children, Gou et al. reported a significant association between VDD and TB with a pooled OR of 1.78 (95% CI: 1.30–2.44, p < 0.05) and between latent tuberculosis infection (LTBI) and VDD with a pooled OR of 1.54 (95% CI: 1.12–2.10, p < 0.05) [49]. Although these studies established a relationship between tuberculosis and serum 25(OH)D levels, it remains uncertain whether VDD status increases the risk of developing tuberculosis or if tuberculosis leads to VDD. Recently, a prospective study conducted by Hsu et al. reported that vitamin D deficiency is an independent risk factor for incident active tuberculosis [10]. Huang et al. suggested that VDD is more probably a cause of TB rather than a result of it [48]. In their review, Junaid et al. concluded that VDD is a risk factor for tuberculosis due to vitamin D's well-documented role in the innate immunity pathway [13]. Moreover, Aibana et al. found in their meta-analysis that low serum 25–(OH)D levels increased the risk of progressing to tuberculosis in a dose-dependent manner [50]. Another piece of evidence supporting the association between VDD and TB is the presence of vitamin D receptor (VDR) gene polymorphisms [51-54]. Sutaria et al. found in their pooled analysis of seven studies that only BsmI and FokI VDR polymorphisms significantly affect TB risk. Specifically, both the heterozygous and recessive forms of BsmI, as well as the recessive form of FokI, are associated with an increased risk of TB compared to the wild-type (ORs of 1.70, 1.55, and 1.93, respectively, p < 0.05) [41]. In their meta-analysis, Huang et al. demonstrate that the VDR FokI polymorphism plays a role in increasing the risk of TB [55]. The positive effects of vitamin D have long been recognized, whether through the administration of cod liver oil, exposure to sunlight, or with proper nutrition and fresh air [13]. In vitro studies demonstrated that vitamin D has the ability to restore impaired immune responses in macrophages exposed to Mycobacterium tuberculosis [56], and the administration of 1,25-dihydroxyvitamin D3 reduced the proliferation of Mycobacterium tuberculosis bacilli inside macrophages [14, 57, 58]. Additionally, low serum levels of 25(OH)D were found to correlate with an increased incidence of upper respiratory tract infections, chronic and acute airway diseases [59, 60], mortality in adult patients with sepsis [61], and many other diseases [62]. Several research into vitamin D as an adjunct treatment for tuberculosis have produced conflicting results in the various clinical trials. Some Randomized Controlled Trials (RCT) studies have confirmed that vitamin D supplementation has positive effects on tuberculosis, including improved therapeutic outcomes [63], accelerated sputum conversion [64], and alleviation of clinical signs of TB [64-66]. In contrast, other studies have reported no effect of vitamin D on reducing the risk of infection, mortality, or acute respiratory infection [67, 68]. A recent review of clinical trials by Santos-Mena et al. highlights that factors such as genetic variations, geographical differences, variable dosages, duration of treatment, tuberculosis status, and the different forms of vitamin D used contribute to the variability observed in results [56].
4.1. Limitations of the Study
Our study is subject to a few limitations. Firstly, we only had access to four electronic databases (PubMed, Scopus, ScienceDirect, and Web of Science), which may have led to publication bias by restricting the diversity of included studies. Secondly, the limited number of studies and small sample sizes may have reduced the reliability of our findings. Thirdly, variations in analytical methods, such as RIA, ELISA, LC-MS/MS, and ECLIA, could have influenced the comparability and accuracy of the findings. Nonetheless, despite the inclusion of various study designs (case-control, cross-sectional, and cohort), Egger's test did not indicate any significant heterogeneity. Additionally, while we focused solely on HIV-negative patients to reduce a key confounding factor, other potential confounders-such as socioeconomic status, nutritional factors, and comorbid conditions-were not systematically addressed. Given the multifactorial nature of TB risk, future studies should incorporate a more comprehensive adjustment for these factors. Despite these limitations, our study demonstrates a link between vitamin D deficiency (VDD) and tuberculosis by consistently defining VDD as 20 ng/mL, which helped maintain uniformity in the findings. Furthermore, our results highlight the need for standardized methodologies and diagnostic criteria in future research to enhance comparability and reliability.
CONCLUSION
Our meta-analysis revealed that tuberculosis patients have significantly lower 25(OH)D levels than controls. Vitamin D deficiency is also significantly associated with an increased risk of TB. These findings highlight the potential role of vitamin D in TB risk and underscore the need for further investigation into its implications for tuberculosis prevention and management. Future research should prioritize standardized vitamin D supplementation trials to assess its effectiveness in TB prevention and treatment. Additionally, developing targeted screening strategies for at-risk populations, such as individuals with chronic vitamin D deficiency or those in high-burden TB areas, could help identify vulnerable groups and guide preventive interventions. Integrating vitamin D assessment into TB management protocols may also provide valuable insights into its role in disease progression and treatment outcomes. While further evidence is necessary before establishing clinical guidelines, these considerations could contribute to a more comprehensive approach to TB control and prevention. Moreover, the standardization of clinical trial protocols on vitamin D supplementation is essential to minimize confounding factors and ensure more conclusive and reproducible results.
AUTHORS’ CONTRIBUTION
The authors confirm their contribution to the paper as follows: conceptualization, methodology, software, investigation, writing - original draft, writing – review: AO; data collection: FZEM; data analysis or interpretation: AL; methodology: AM; methodology, validation, writing - review & editing: SM; conceptualization, methodology, supervision, project administration, writing - review & editing: SB. All authors reviewed the results and approved the final version of the manuscript.
AVAILABILITY OF DATA AND MATERIAL
All data generated or analyzed during this study are included in this published article.
ACKNOWLEDGEMENTS
I would like to thank all the authors who contributed to this article.